The degeneration of axons is a widespread event in the developing nervous system, and also considered to be a hallmark of nervous system injury and neurodegenerative disease. Remarkably, the underlying molecular mechanisms that execute axon degeneration remain poorly understood in any context, making the development of efficacious treatments to block axon loss challenging.
We are interested in identifying genes required for injury-induced axon degeneration (axon death). It is important to delineate axon death signaling, because it is a shared pathway in injury and disease, and conserved throughout evolution. Injury activates axon death signaling in the distal, from the soma separated axon. While severed wild type axons undergo axon death within 1 day, and resulting debris is cleared within 3-5 days, axons defective in axon death remain preserved for the life span of the fly, and weeks in mice. We study axon death signaling in Drosophila, and are able to use highly sophisticated tools with which to observe morphology and function of axons and their synapses:
Morphology: Axon death can be observed in injured axons side-by-side of uninjured controls of sensory neurons in the same nerve bundle in the wing. It allows for visual resolution with previously unprecedented depth.
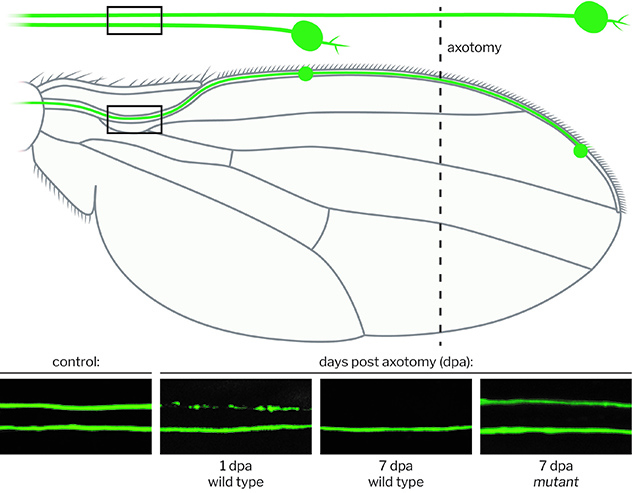
Function: The functional preservation of severed, axon death defective axons and their synapses can be measured in specific sensory neurons required and sufficient for antennal grooming. Antennal ablation results in the removal of somas, whereas axons & synapse remain in the CNS: they are activated by optogenetics, and the resulting antennal grooming behavior serves as an in vivo readout for functional preservation.
Optogenetics combined with grooming as a behavioral readout to measure functional preservation of axons and their synapses.